Magnetic saturation
What is magnetic saturation?
When a ferromagnetic object is magnetised, the magnetic forces initially increase in proportion to the strength of the magnetising field. At some point, however, saturation is reached and the magnetic forces hardly increase at all. This effect is called magnetic saturation and is the reason for the limited strength of permanent magnets. Because of magnetic saturation, there can be no arbitrarily strong magnets.Table of Contents
Physics explanation
Saturation magnetisation is the maximum possible magnetisation of a material. This fact is referred to as magnetic saturation. If an attempt is made to increase the magnetisation even further, the material behaves like empty space (vacuum) if the external magnetic field is increased further. Like magnetisation, magnetic saturation can also be observed, particularly in ferromagnetic materials.When a ferromagnet is placed in an external magnetic field H, the magnetic flux density B increases very strongly. This is because the magnetic field aligns the electron spins in the matter of the ferromagnet and the magnetic moments of these spins, i.e. the elementary magnets in the material, are arranged in parallel. This process is also known as magnetic polarisation. It strengthens the external field, resulting in a strong increase in magnetic flux density and of the magnetic field outside the ferromagnet.
At some point, however, all existing spins are aligned in parallel.
When that's the case, no further amplification of the external magnetic field is possible if it is increased even further. The flux density then behaves as if in a vacuum when the external magnetic field is increased further.
Magnetic saturation experiment
Magnetic saturation can be observed in an experiment. You will need a coil with an iron core, an adjustable voltage source and a device for measuring the magnetic flux density, such as a Hall probe. In the experiment, the magnetic flux density on the surface of the iron core is then measured as a function of the applied current. If the current through the iron coil is increased, the magnetic flux density initially increases significantly. If the current is doubled, the flux density also approximately doubles. At a certain point, however, a saturation effect occurs and the magnetic flux density will only increase very slowly. At this point, the magnetic saturation of the iron material is reached. For iron, this is already the case at a flux density of 1–2 tesla.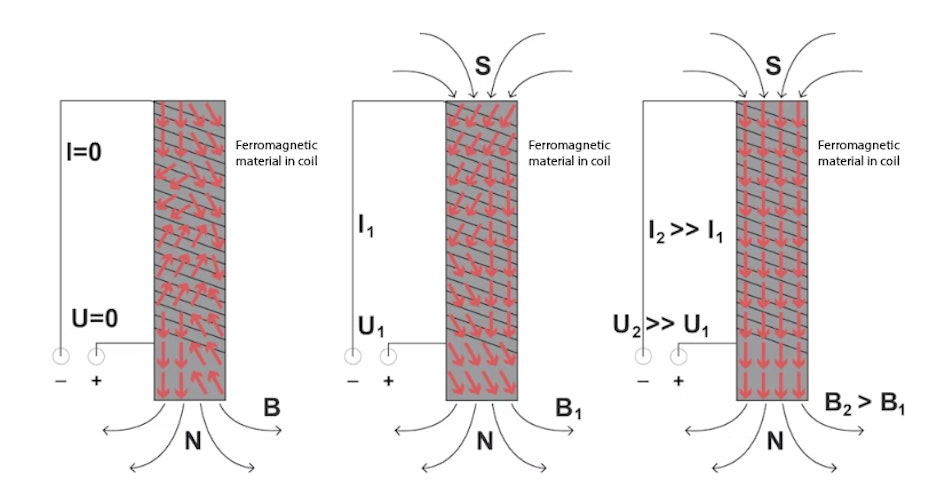
The illustration shows the course of the magnetic flux density B during the magnetisation of a ferromagnetic material in a coil.
Without current I (I =0), i.e.
without potential U (U=0) , the electron spins (shown in red) are only aligned in parallel in the Weiss domains.
Overall, the magnetisation cancels out and is zero in the outer space (left-hand image).
When a current I1
is applied (increasing the potential U to the value U1), the magnetic flux density B rises sharply (to the value B1) because the various Weiss domains merge and more and more atomic spins are aligned in parallel.
If the coil current I is then further increased to the value I2,
which can be much greater than I1,
it can be observed that the magnetic flux density and thus the magnetic forces increase only slightly (to the value B2,
which is only slightly greater than B1).
All spins are then aligned in parallel and a further increase in the current results in only a slight increase in the magnetic forces.
What happens in the range of saturation magnetisation?
In the range of saturation magnetisation, the magnetic permeability μ of the ferromagnet drops sharply and approaches the value μ=1. This also reduces the magnetic conductivity of the ferromagnet to the value of the vacuum.One can think of this as the alignment of the atomic spins resulting in increased conductivity for magnetic fields. Significantly more magnetic field lines "fit" through a ferromagnet than through a vacuum. However, if the field becomes so strong that this increased conductivity is at its maximum, no more field lines will "fit" through the ferromagnet than through the vacuum if the magnetic field is increased further. This happens precisely when saturation magnetisation is reached.
If a ferromagnetic material is exposed to saturation magnetisation, the maximum number of atomic spins is aligned in parallel. The magnetisation does not increase any further. In this case, the highest possible magnetisation also remains when the external field is switched off again (without reversing the polarity). This remaining magnetisation is referred to as remanence.
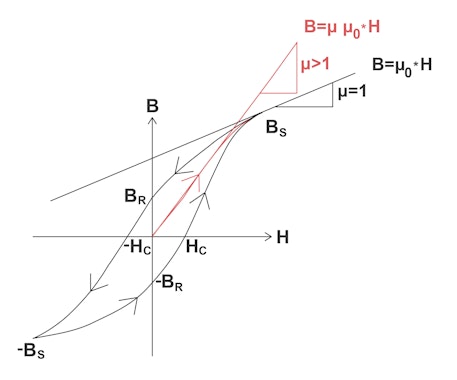
The illustration shows a hysteresis curve of a magnetically hard material, such as that used in transformers.
The point BS
denotes the saturation magnetisation.
Here, the magnetic flux density B increases only slightly with a further increase in the external magnetic field H (the gradient of the black straight line has the value µ0,
as µ=1).
With smaller magnetic fields, the magnetisation increases more strongly by a factor of µ (red straight line).
For iron, µ can assume a value of several thousand.
At the point of saturation magnetisation, µ=1.
The point BR
denotes the remanence that remains when the external field is switched off again after magnetic saturation.
Consequences of saturation magnetisation for technical applications
Saturation magnetisation means that a magnetic field is no longer amplified by a ferromagnetic material.This leads to disadvantages in numerous technical applications. Transformers, for example, are pairs of opposing coils with different numbers of turns. The coils have an iron core, which allows for a very efficient conversion of electrical energy into the magnetic energy of a strong magnetic field within the coil. This magnetic field in turn induces a current in the opposite coil with specific voltage and current values that can be set by the properties of this coil, though with similar total power.
When the iron core has a high magnetic permeability μ, this method of converting the electrical voltage is very efficient. Little power is lost. For example, the transformer can reduce the socket voltage from 220 V to 12 V without any major loss because the current in the primary circuit is correspondingly low. In the range of saturation magnetisation, the efficiency of converting electrical energy into magnetic energy decreases, and thus the efficiency of the transformer. Saturation magnetisation is therefore undesirable in transformers.
An air gap prevents premature magnetic saturation
By cutting an air gap into the iron core, the increase in magnetic flux density can be slowed down and magnetic saturation occurs later. The air gap has a much higher magnetic resistance than the iron core. Consequently, it impedes the magnetisation of the ferromagnetic material. Overall, the magnetisation of the transformer core therefore increases more slowly than it would without an air gap. But, on the other hand, the magnetisation is proportional to the external field over a wide range.That is why an air gap has a truly technical significance in magnetic field technology.
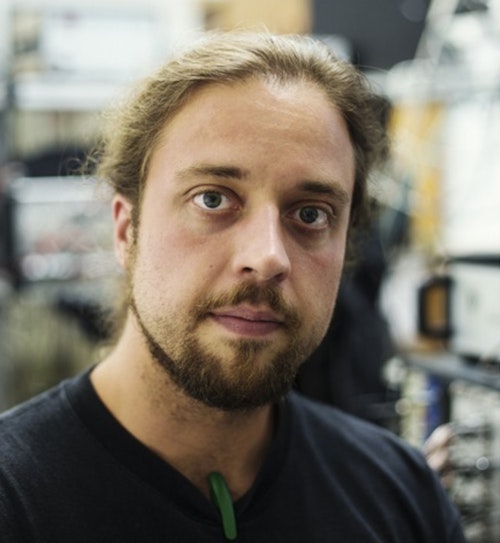
Author:
Dr Franz-Josef Schmitt
Dr Franz-Josef Schmitt is a physicist and academic director of the advanced practicum in physics at Martin Luther University Halle-Wittenberg. He worked at the Technical University from 2011-2019, heading various teaching projects and the chemistry project laboratory. His research focus is time-resolved fluorescence spectroscopy in biologically active macromolecules. He is also the Managing Director of Sensoik Technologies GmbH.
Dr Franz-Josef Schmitt
Dr Franz-Josef Schmitt is a physicist and academic director of the advanced practicum in physics at Martin Luther University Halle-Wittenberg. He worked at the Technical University from 2011-2019, heading various teaching projects and the chemistry project laboratory. His research focus is time-resolved fluorescence spectroscopy in biologically active macromolecules. He is also the Managing Director of Sensoik Technologies GmbH.
The copyright for all content in this compendium (text, photos, illustrations, etc.) remains with the author, Franz-Josef Schmitt. The exclusive rights of use for this work remain with Webcraft GmbH, Switzerland (as the operator of supermagnete.ro). Without the explicit permission of Webcraft GmbH, the contents of this compendium may neither be copied nor used for any other purpose. Suggestions to improve or praise for the quality of the work should be sent via e-mail to
[email protected]
© 2008-2025 Webcraft GmbH
© 2008-2025 Webcraft GmbH